The cause of high Tc superconductivity at the interface between FeSe and SrTiO3
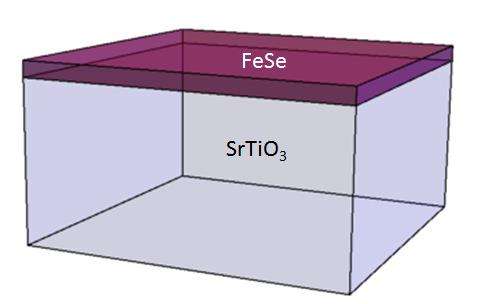
The temperature above which a superconductor turns into a normal conductor is called the superconducting transition temperature. Raising it to a point enabling practical applications is a dream in modern science and technology. In 1987, a superconductor with a transition temperature above the boiling point of liquid nitrogen was discovered. Today, several families of closely related superconducting compounds (some with even higher transition temperatures) are known. They are called the "cuprates," as they're built from copper oxides.
In 2006, another wave of excitement hit the scientific community after the discovery of a family of high-temperature superconducting compounds. The building blocks for this family are iron-pnictides (pnictogens are members of group V elements of the periodic table). Recent developments have raised the superconducting transition temperature to the boiling point of liquid nitrogen.
In the superconducting state, electrons bind together to form pairs. They are called "Cooper pairs," named after Leon Cooper, who first theorized their existence. These pairs are the current carriers in a superconductor. The binding energy of Cooper pairs is a measure of the robustness of the superconducting state—larger binding energy implies higher superconducting transition temperature. In 2012, the binding energy of an anomalously large Cooper pair was observed by a research team lead by Qi-Kun Xue at Tsinghua University in Beijing at the interface between an atomically thin iron selenide (FeSe) film grown on the strontium titanate (SrTiO3) substrate (see Fig.1). In the literature, such an interface is abbreviated as FeSe/SrTiO3. The value of the binding energy suggests that the superconducting critical temperature, Tc, can exceed the boiling point of liquid nitrogen.
Later, an "angle resolved photoemission spectroscopy" experiment led by Zhi-Xun Shen at Stanford provided compelling evidence that the electrons in the FeSe film couple strongly with the atomic vibrations in SrTiO3, hence raising the intriguing possibility that such coupling could be responsible for the high superconducting transition temperature.
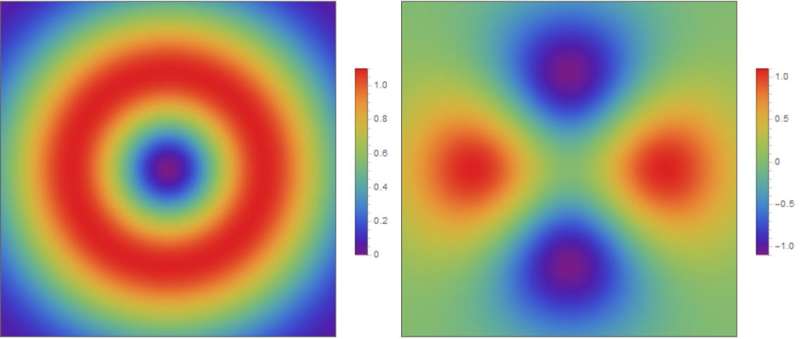
However, subsequent developments demonstrate that aside from the above electron-phonon coupling, there is another factor contributing to the high Tc of FeSe/SrTiO3. For systems composed of FeSe building blocks but free of the SrTiO3 substrate, Tc can also be significantly raised by injecting extra electrons into the system, a process referred to as "electron doping." (Incidentally, it turns out the FeSe in FeSe/SrTiO3 is also electron doped.) However, compared with the critical temperature of FeSe/SrTiO3, the Tc of those without SrTiO3 substrate is considerably lower. Thus, scientists are also seeking the causes the high Tc in systems with electron doping but without SrTiO3.
It turns out both copper oxides and iron-based superconductors are "strongly correlated" electron systems. In theory, "strong correlation" is like a cursed phrase. The reality is far from the easily understood ideal limits. Thus, it is hopeless to try to understand the real situation based on the knowledge of simple limits. This is partly why the mechanism that causes the large pair binding energy in the copper-oxide superconductors is still an unsolved problem.
A numerical technique known as the "quantum Monte Carlo simulation" allows the study of strongly correlated systems by brute force. However, for most realistic situations, this method is plagued with the famous "fermion sign problem," which is ultimately associated with the Fermi statistics of electrons—specifically, the quantum mechanical wave function changes sign when two electrons are exchanged. In the presence of the minus-sign problem, it is practically impossible to reliably compute the low temperature properties of a system consisting of a large number of elections. Unfortunately, the fermion sign problem presents for strongly correlated electron problems unless there is a symmetry to ensure that minus signs pair up to yield plus signs, e.g.,. -1 × -1 = + 1.
![The phase diagram deduced in Ref.[1]. The black symbols mark the phase boundary of the 'antiferromagnetic' order and the red ones mark the superconducting transition. The open and solid red circles refer to the presence and absence of electron-phonon interaction. In the absence of SrTiO3 it is a magnetic interaction that causes the formation of Cooper pairs. Credit: ©Science China Press The cause of high Tc superconductivity at the interface between FeSe and SrTiO3](https://scx1.b-cdn.net/csz/news/800a/2016/2-thecauseofhi.jpg)
What is realized in Ref. 1 is that such a symmetry exists for the problem of FeSe/SrTiO3. Thus, Li et al. were able to examine a number of electron-electron and electron-phonon interactions that are potentially responsible for causing the formation of Cooper pairs. (Figuring out which interaction is responsible for the pairing of electrons is referred to as the "pairing mechanism problem.")
In particular, they were able to determine the size of the binding energy and the symmetry of pair wave functions (see Fig.2). The Cooper pair wave function is very similar to the molecular wave function for, say, H2. It is the quantum mechanical wave function of a Cooper pair as a function of the relative coordinates between the two electrons. The symmetry of the pair wave function refers to its phase behavior upon rotating the relative coordinate. With the calculated binding energy and symmetry of the pair wave function, Li et al. referred to experiments and suggested the most likely interaction that triggers superconductivity in the absence of the SrTiO3 substrate.
In the presence of the SrTiO3 substrate, they determined the enhancement of the pair binding energy by the interaction between the FeSe electron and SrTiO3 phonons and confirmed that the interaction substantially raises Tc. Based on these results, they constructed a "phase diagram" like the one in Fig.3. There, the difference between the red solid circles and open circles reflects the Tc enhancement by the substrate phonons.
In addition to its importance to the understanding of high Tc in FeSe/StTiO3, these results also point out two separate but cooperative mechanisms driving high temperature superconductivity. Hopefully, it will provide hints to other higher-temperature superconducting materials. The researchers believe they have performed "the first numerically exact, sign-problem-free quantum Monte Carlo simulations to study the pairing mechanism in iron-based superconductors."
More information: Zi-Xiang Li et al, What makes the T c of monolayer FeSe on SrTiO3 so high: a sign-problem-free quantum Monte Carlo study, Science Bulletin (2016). DOI: 10.1007/s11434-016-1087-x
Provided by Science China Press